16.03.2023
The rise of PLA: is it here to stay?
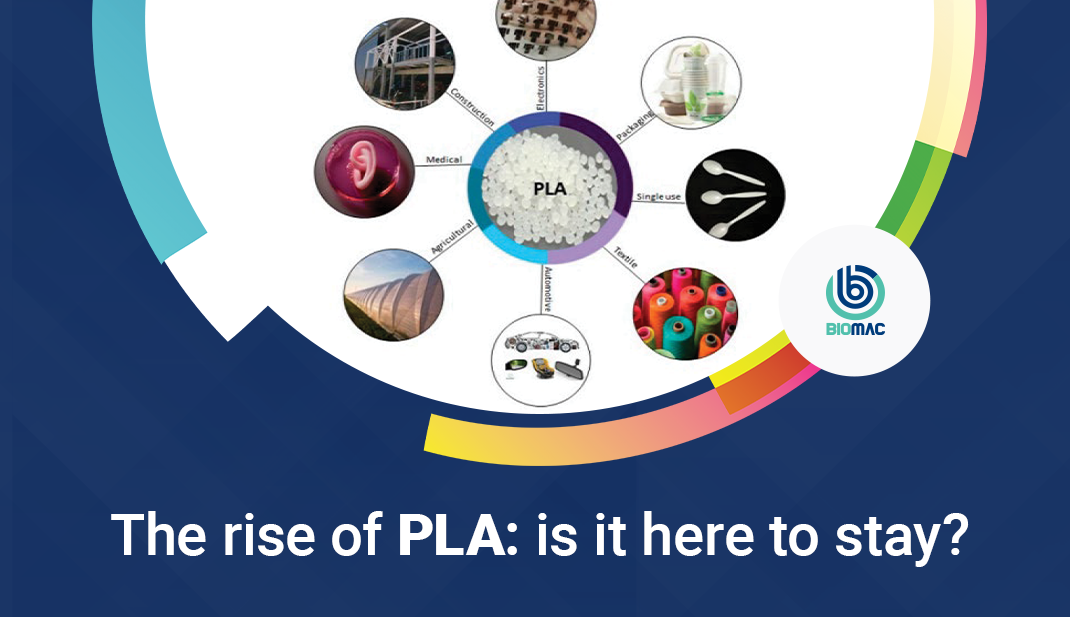
One of the most cardinal issues the academic community and industry has to face is the establishment of polymers that are derived from renewable resources among the global market of polymers derived from petrochemicals. Unfortunately, the production capacities ratio of bioplastics to plastic is still insufficient. Besides the insignificant global production capacities of bioplastics in 2022, their total amount until 2027 based on statistical predictions, will be increased approximately three times (6.3 million tons). Figure 1 illustrates the global production capacities of bioplastics for 2027 and 2022 and poly(lactic acid) (PLA) accounts for the largest portion of global production capacities of bioplastics. Without doubt can be considered one of the most promising biobased polyester of the 21th century [1].
PLA has gained the attention of academia not only by its remarkable applicability and versatility but for its fully bio-based nature. It is a commercially available product which is mostly made of from cellulose, starch, corn and waste materials and products. The main production route of PLA is fermentation from starch [2] to lactic acid to produce lactide and its polymerization (via polycondensation, azeotropic dehydrative condensation or Ring-Opening-Polymerization – ROP [3]). It should be noticed it is the only polymer among the mass-produced which is biocompatible, compostable, and bio-based at the same time [4, 5]. Moreover, it is an excellent example of an eco-friendly polymer to substitute traditional ones, because during its production the consumption energy and the greenhouse gases are reduced more than 60 and 65 %, respectively. A key innovation in the polymer industry is the development of biodegradable polymers that address environmental issues. As a result, PLA is used in plethora of applications, such as in the sector of automobile, in biomedical applications, as textiles, material for packaging applications, for construction to agriculture (Figure 2) [5,6].
Figure 1: Global production capacities of bioplastics for a) 2027 and b) 2022 [1].
Figure 2: Applications of PLA [5].
The need of an alternative and unique material
The great importance of PLA relies on its applicability at several fields. For instance, it can be used as an implant material for surgeries, for drug delivery systems and as a scaffold for tissue engineering [7]. Unlike polymer implants that remain in the body until they are degraded and then need to be removed once the defect sites have been repaired, biodegradable polymer implants do not need a secondary operation to be removed, so the importance of PLA in the medical field is unprecedented, considering its non-toxic and safe nature [8]. An extremely groundbreaking field which combines the needs of efficiency and reliability and fulfills the purpose of great ideas is the technology of 3D-printing. Three dimensional structures can be prepared and for example, PLA-based nanocomposites with bioactive coatings could be used as an alternative substrate for tissue engineering in comparison with neat materials [9]. Studies have shown that PLA-based micro- and nano- composites are suitable materials for targeted delivery of bioactive compounds satisfying the needs of the pharmaceutical sector [10,11]. In addition to medical applications, PLA could be utilized to treat blood vessels, organs, to regenerate skin and for the treatment of cancer [5].
PLA is an aliphatic linear crystallizable thermoplastic polyester with exceptional properties for packaging applications too. In comparison to most thermoplastic fossil-based polyesters, PLA has comparable or even superior thermal properties. The glass transition temperature (Tg) and melting temperature (Tm) can vary between 50 – 60 oC and 160 – 180 oC and depend on its molecular weight and optical purity. Most disposable cups used to be made of polypropylene (PP) or poly(ethylene terephthalate) (PET), but because the Tg of PLA fluctuates between the PP’s and PET’s Tg and its Tm is high enough to be an affordable material for industrial purposes, it is already established in the market of food packaging, by combining of course its biocompatible features [12, 13].
One of the restrictions of PLA that must be surpassed is its unsatisfying mechanical performance. It can be considered as a tough material with an Elastic Modulus (E) around 3-4 GPa and a tensile strength (σ) at 50-70 MPa, but unfortunately, it’s a brittle material with an elongation of 10%. The inadequate gas barrier properties of PLA pose a risk for packaging application and for example the replacement of PET which exhibits appropriate gas barrier features [14]. One of the most important fashions to deal with those drawbacks is to synthesize novel complex material based on PLA. Copolymerization can be used as an efficient way to enhance the tensile properties of PLA. Aliphatic polyesters have shown to be an excellent example and particularly poly(lactic acid)-co-poly(propylene adipate) block copolymers exhibit elongation around 110% [15]. PLA/lignin composites are another example of novel materials with enhanced mechanical and antioxidant properties [16,17]. Furthermore, given that PLA textiles are now readily commercial and have sufficient qualities that make it suitable for industrial processing, the replacement of PET in the fiber sector is a likely future outcome [14]. Generally, a plethora of new composites based on PLA have been prepared to investigate antibacterial, thermal, mechanical and barrier properties. Some examples are PLA composites based on lignin films, ZnO membranes, halloysite nanotubes bionanocomposites or cinnamaldehyde inclusions films [5].
Taking into consideration the versatility of PLA, not only in the next years its production will grow exponentially, but also PLA will substitute most of the current thermoplastics derived from non-renewable resources. it should be noted that the number of publications have been increased dramatically, since the first one that was reported in 1984. In 2020 the outstanding number of at least 4000 published papers based on PLA have been reported [18]. People's awareness has increased regarding the safety of the earth’s ecosystems and the consequences of using materials that could be hazardous to them have led to alternative options for the establishment of sustainability [5].
The ability to meet the demands of the present generation without compromising the capacity of future generations to meet their own needs is referred as the sustainability. The most well-known component of sustainability, environmental sustainability, refers to the responsibility to maintain the environment and preserve resources for future generations. This can involve enhancing sustainable agriculture, conserving biodiversity, and limiting on carbon emissions. An integrated effort from people, corporations, governments, and civil society organizations is necessary for a sustainable development. A material that can be a part of this challenging but important development toward a better and safer future is PLA [2].
Properties of nanocellulose reinforced polymers
The addition of nanocellulose leads to improvement in overall performance of polymer composites by improving their mechanical, thermal and barrier properties, usually even at very low content.
Production and applications of PLA in the BIOMAC project
In BIOMAC, PLA is produced starting from the fermentation of biomass to receive its monomer, L-lactic acid, in
Pilot Line 7 by Enzymatic Hydrolysis & Microbial Fermentation. Then, lactic acid is transformed to lactide which is polymerized to poly(lactic acid) with reactive extrusion (REX), a novel, fast and efficient process in less than 15 min. AIMPLAS with
Pilot Line 11 can synthesize not only PLA but also its copolymers and nanocomposites. Copolymerization is necessary to improve the elasticity of PLA and increase its hydrolytic degradation rate.
PLA-based products will be demonstrated in the internal Test Cases of BIOMAC. In
Test Case 2, NOVAMONT will test PLA-based mulching and grow pots for agricultural applications. In
Test Case 3, EVERSIA will use PLA-based films for 100% biodegradable, compostable and self-cleaning food packaging applications.
Are you interested to upscale your novel PLA concept free of charge?
References
[1]
https://www.european-bioplastics.org/market/#.
[2]
Zhu Y, Romain C, Williams CK. Sustainable polymers from renewable resources. Nature. 2016;540(7633):354-362. doi:10.1038/nature21001
[3]
Lopes MS, Jardini AL, Filho RM. Synthesis and characterizations of poly (lactic acid) by ring-opening polymerization for biomedical applications. Chem Eng Trans. 2014;38:331-336. doi:10.3303/CET1438056
[4]
de França JOC, da Silva Valadares D, Paiva MF, Dias SCL, Dias JA. Polymers Based on PLA from Synthesis Using D,L-Lactic Acid (or Racemic Lactide) and Some Biomedical Applications: A Short Review. Polymers. 2022;14(12):2317. doi:10.3390/polym14122317
[5]
Balla E, Daniilidis V, Karlioti G, et al. Poly(lactic acid): A versatile biobased polymer for the future with multifunctional properties-from monomer synthesis, polymerization techniques and molecular weight increase to PLA applications. Polymers. 2021;13(11):1822. doi:10.3390/polym13111822
[6]
Shen L, Worrell E, Patel MK. Comparing life cycle energy and GHG emissions of bio-based PET, recycled PET, PLA, and man-made cellulosics. Biofuels, Bioproducts and Biorefining. 2012;6(6):625-639. doi:10.1002/bbb.1368
[7]
Liu S, Qin S, He M, Zhou D, Qin Q, Wang H. Current applications of poly(lactic acid) composites in tissue engineering and drug delivery. Compos B Eng. 2020;199,(2):108238. doi:10.1016/j.compositesb.2020.108238
[8]
Lasprilla AJR, Martinez GAR, Lunelli BH, Jardini AL, Filho RM. Poly-lactic acid synthesis for application in biomedical devices - A review. Biotechnol Adv. 2012;30(1):321-328. doi:10.1016/j.biotechadv.2011.06.019
[9]
Grigora ME, Terzopoulou Z, Baciu D, et al. 3D printed poly(lactic acid)-based nanocomposite scaffolds with bioactive coatings for tissue engineering applications. J Mater Sci. 2023;58(6). doi:10.1007/s10853-023-08149-4
[10]
Singhvi MS, Zinjarde SS, Gokhale D v. Polylactic acid: synthesis and biomedical applications. J Appl Microbiol. 2019;127(6):1612-1626. doi:10.1111/jam.14290
[11]
Vlachopoulos A, Karlioti G, Balla E, et al. Poly(Lactic Acid)-Based Microparticles for Drug Delivery Applications: An Overview of Recent Advances. Pharmaceutics. 2022;14(2):359. doi:10.3390/pharmaceutics14020359
[12]
Saeidlou S, Huneault MA, Li H, Park CB. Poly(lactic acid) crystallization. Prog Polym Sci. 2012;37(12):1657-1677. doi:10.1016/j.progpolymsci.2012.07.005
[13]
Lim LT, Auras R, Rubino M. Processing technologies for poly(lactic acid). Prog Polym Sci. 2008;33(8):820-852. doi:10.1016/j.progpolymsci.2008.05.004
[14]
Sousa AF, Patrício R, Terzopoulou Z, et al. Recommendations for replacing PET on packaging, fiber, and film materials with biobased counterparts. Green Chemistry. 2021;23(22):8795-8820. doi:10.1039/d1gc02082j
[15]
Terzopoulou Z, Zamboulis A, Bikiaris DN, Valera MA, Mangas A. Synthesis, properties, and enzymatic hydrolysis of poly(Lactic acid)-co-poly(propylene adipate) block copolymers prepared by reactive extrusion. Polymers. 2021;13(23):4121. doi:10.3390/polym13234121
[16]
Makri SP, Xanthopoulou E, Klonos PA, et al. Effect of Micro- and Nano-Lignin on the Thermal, Mechanical, and Antioxidant Properties of Biobased PLA–Lignin Composite Films. Polymers. 2022;14(23):5274. doi:10.3390/polym14235274
[17]
Terzopoulou Z, Xanthopoulou E, Pardalis N, et al. Synthesis and Characterization of Poly(lactic acid) Composites with Organosolv Lignin. Molecules. 2022;27(23):8143. doi:10.3390/molecules27238143
[18]
Auras R, Lim LT, Selke SEM, Tsuji H. Poly(Lactic Acid): Synthesis, Structures, Properties, Processing, Applications, and End of Life.; 2022.